«After days and nights of incredible labour and fatigue, I succeeded in discovering the cause of […] life; nay, more, I became myself capable of bestowing animation upon lifeless matter».
—Frankenstein or the Modern Prometheus, Mary Shelley (1818)
In 2018, we will celebrate 200 years of the first edition of “Frankenstein, or the modern Prometheus”, the fruit of the genius and creativity of a young Mary Shelley [1]. In her book, influenced by Galvani and Volta’s contemporary electrophysiology experiments, she suggested endowing lifeless matter with “vital warmth” or “a spark of life”, creating the myth of Frankenstein we know today.
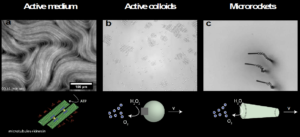
Figure 1 Synthetic active matter. a) Active medium composed of microtubules coupled with molecular motors and fuelled by ATP [4]. b) Micron-sized catalytic particles self-propel undergo a phase transition and form flocks when UV light is on [5]. c) Microrockets self-propelling by decomposition of hydrogen peroxide [6].
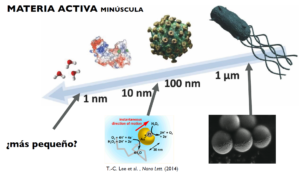
Figure 2 Illustration of natural and synthetic active matter at the nanoscale. On the top, from right to left (bacterium, virus, protein and molecules). On the bottom, chemically propelled Janus micromotors and nanomotors.
Although not everything that moves is alive, it could still be active matter if it moves autonomously and consumes energy. A rock is not active. Pollen particles diffuse in water, and still, are not active. But a bacterium is active. A new research area has emerged at the interface of the physical and biological sciences that studies active matter. Active systems remain out of thermodynamic equilibrium by consuming energy in the environment (or internally stored) to move. Far from boring thermodynamic equilibrium (this is a state where things don’t change, i.e. no flow of matter or energy), active particles interact with each other to form new collective phenomena that we still do not understand. Active systems are around us: from flocks of birds, school of fish or bacteria colonies, to humans. One of the astonishing properties of active materials is that they exhibit unexpected features when they consume energy and this occurs at different length scales, e.g. leaderless birds form sudden synchronised flocks in the sky and cytoskeletons self-assemble complex structures in the cell.
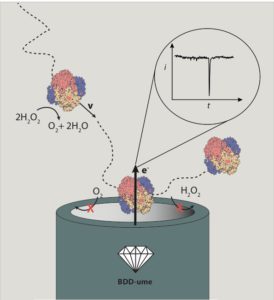
Figure 3 Detection of enzymatic nanomotors (catalase) on the surface of an ultramicroelectrode. The dashed lines correspond to a trajectory. A collision (known as nanoimpact) is identified as a peak in the detected current [7].
Another important class of active matter systems is that of chemically active particles. These synthetic particles achieve self-propelled motion via the catalytic reaction of a fuel in their environment. A notable example (shown in Figure 1.b) developed in New York University (NYU) consists of asymmetric particles of haematite, an iron oxide mineral, inside a spherical polymer (the name of these particles is Janus, like the Roman two-faced god) [5]. In the presence of fuel and some light, these particles catalyse a reaction causing the particles to move around spontaneously, forming groups of particles like a flock of birds in the sky. Without fuel or light, particles stop their activity and ungroup. These “living particles” have opened up a new research area in materials science, and have inspired many other types of “swimming” particles, such as the micro rockets in Figure 1.c [6].
But how small can self-propelled particles be? And how can we observe them at the nanoscale? These are exciting open scientific questions. So far, the scientific community has achieved synthetic nanomotors of several tens of nanometres (the thickness of a hair is approximately 100,000 nanometres). In our laboratory, we have developed new ways to make even smaller nanomotors (Figure 2). Also, there are many enzymes smaller than 10 nm that exhibit self-propulsion properties, such as catalase and urease. Metallic and enzymatic nanomotors are examples of active matter at the nanoscale and we measure their mobility by fluorescence microscopy or more recently, by using tiny electrodes (Figure 3) [7]. Active matter at the nanoscale has potential applications in active transport on small scales, such as for drug delivery in nanomedicine. Chemically-propelled nanomotors could give new insight into locomotion and self-organisation of living organisms and help discover new ways of converting energy into motion yet unseen in nature. As more researchers delve into active matter physics, we will be able to discover new rules that govern the behaviour of these systems, and, who knows, we could be closer to Shelley’s dream of bringing a spark of life to matter.
By Dr. Ibón Santiago. Postdoctoral Research Assistant at the University of Oxford. SRUK Oxford Constituency.
More information in:
Webpage: https://www2.physics.ox.ac.uk/contacts/people/santiago
[1] M. Shelley, Frankenstein: Annotated for Scientists, Engineers, and Creators of All Kinds -MIT Press (2017).
–Free open access click here
-Global Frankenstein Bicentennial Project (here)
[2] R. Brown, A Brief Account of Microscopical Observations … on the Particles Contained in the Pollen of Plants; and on the General Existence of Active Molecules in Organic and Inorganic Bodies. 1828. Access here
[3] A. Einstein, “On the motion of small particles suspended in liquids at rest required by the molecular-kinetic theory of heat”, Annalen Der Physik 17 (1905). Access here
.
[4] T. Sanchez, D. T. N. Chen, S. J. DeCamp, M. Heymann, and Z. Dogic, “Spontaneous motion in hierarchically assembled active matter,” Nature, vol. 491, no. 7424, pp. 431–+, 2012. Access here
[5] J. Palacci, S. Sacanna, A. P. Steinberg, D. J. Pine, and P. M. Chaikin, “Living Crystals of Light-Activated Colloidal Surfers,” Science, vol. 339, no. 6122, pp. 936–940, 2013. Access here
[6] Li, Jinxing, Isaac Rozen, and Joseph Wang. “Rocket science at the nanoscale.” ACS nano 10.6 (2016): 5619-5634. Access here
[7] L. Jiang*, I. Santiago*, and J. Foord, “Observation of nanoimpact events of catalase on diamond ultramicroelectrodes by direct electron transfer,” Chem. Commun., vol. 53, no. 59, pp. 8332–8335, Jul. 2017. Access here