Looking back, I still remember a famous series of cartoons called “Once upon a time: Life “. There, they tried to explain to kids (but not only) in a very simple way, the mechanisms that regulate the good functioning of the organism. One of those episodes talked about how our brain is able to send messages to the rest of the body thanks to certain “tiny creatures ” that carried a message and ran at high speed. Later on we understood that those “tiny creatures” were nothing more than the way to explain the nervous transmission.
We know that neurons are the basic units on this communication. However, it is difficult to understand the reason why this conversation between the brain and the human body happens. Following this idea, what do you think if I tell you that the key to all this communication between neurons is pure electricity? Probably, the first thing that could come to your mind is the image of Dr Frankenstein giving life to his “monster” through the electrical power of lightning discharge from a storm. Well, Mary Shelley was not very misguided.
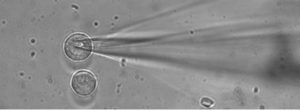
Patch-clamp technique and seal formation on a mouse Chromaffin cell, using a patch-clamp pipette
There is a type of cells that we call “excitable” (such as neurons or chromaffin cells from the adrenal gland, among others). They received that name because they can be electrically stimulated. Galvani or Volta were the first scientists in the eighteenth century describing “animal electricity” thanks to an experiment where they showed the contraction of frog legs’ muscles by applying an electrical stimulus to their nerves. It was later, in the nineteenth century, when the German scientist du Bois-Reymond took these theories and demonstrated the “electrical nature of nerve signaling“, findings that derived into the development of Electrophysiology, the science studying the electrical nature of excitable cells. Julius Bernstein studies helped to measure for the first time the momentary electrical changes in the surfaces of the cells that give rise to this communication: the”action potential”. In 1930, Curtis and Cole measured the action potentials using the axon (one of the parts of the neuron) of the giant squid (as these neurons are extremely big compared to the neurosn in other organisms) by inserting electrodes in two points of the axon and measuring electrical exchanges or electric potential.
These excitable cells separate charges on both sides of the cell membrane, generating electrical potential, like an electrical capacitator. However, these membranes are not “pure” capacitors, as they contain “holes” known as ion channels, which help the exchange of substances between the outside and inside of the cells. An example of these substances are ions such as sodium, potassium and calcium. Doctors Neher and Sackman, who created the patch clamp, one of the most used electrophysiological techniques nowadays, confirmed the existence of ion channels in 1970. Both scientists received the Nobel Prize in Physiology or Medicine in 1991.
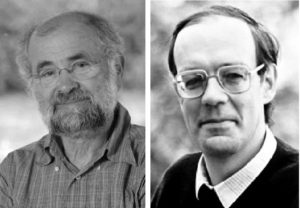
Professors Erwin Neher and Bert Sackman, Nobel prize (1991)
The patch-clamp technique, uses a glass microelectrode connected to the cell membrane of our interest. This strong connection, called Seal or Gigaseal, offers an electric resistance of the order one billion of ohms (unit to measure electrical resistance) and reduces the interference of any external electrical signal, generating a great stability for the study of various electrophysiological factors in the cell such as the movement of ions through the channels already mentioned.
The patch-clamp technique offers different configurations. As an example, we can mention the “whole cell” and the “perforated patch”, allowing both of them a whole connection with the cell in order to detect any electrical change occurring on it. Changes can also be detected only in a small area of the cell membrane and in that case, the cell configuration is known as “cell-attached”. These patch clamp configurations, among others, allow to measure very small electrical changes. As an example of this are the changes with value of a trillionth part of an Ampere, if we speak of electric current.
Electrophysiology and patch-clamp help not only to understand the mechanisms of ion channels, but also to identify differences in their function. For example, if we work with cells from a certain disease (with changes in their structure or mutations).
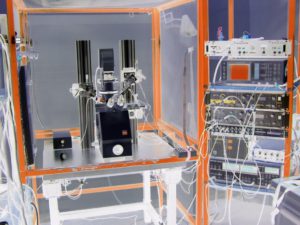
Electrophysiology rig
Throughout my career as electrophysiologist, I have worked on different research projects related to the study of neurosecretion and neurodegenerative diseases. Thanks to the use of this technique we have been able to understand the differences between several ion channels from these excitable cells which sometimes may contain mutations related to diseases such as Alzheimer’s or Amyotrophic Lateral Sclerosis (ALS). In addition, we have been able to confirm the maturity stage of neurons derived from stem cells. All this analysis verifies if the cells comply with the standard of the primary neurons and they maintain the corresponding electrophysiological characteristics. This helps to ensure the reliability of the cellular models used to study different diseases.
Electrophysiology is key in the scientific understanding of diseases, the characterization of cellular models and the mechanisms underlying the physiology of excitable cells. Thanks to the development of these techniques, which may seem basic, we are able to understand the rules that lead those “tiny beings” to carry messages quickly between the brain and the rest of the human body. In addition, all this knowledge brings a better understanding of the mechanisms related to different diseases, the first step to be able to fight them.
By Dr. Margarita Segovia Roldán. Postdoctoral Researcher at the University of Sheffield. Yorkshire SRUK Constituency
More information:
https://en.wikipedia.org/wiki/Erwin_Neher
http://www.pnas.org/content/107/44/19032
https://link.springer.com/article/10.1007%2Fs00424-006-0169-z
Electronics for electrophysiologists
Electronics for electrophysiologists II