Cardiovascular diseases are one of the main causes of death in industrialized societies. In addition, a large number of these deaths are related to arrhythmias, which are caused by an abnormal electrical functioning of the heart [1]. Among the main factors that influence the development of arrhythmias are the existence of cardiac tissue with a tendency to develop arrhythmias, a specific triggering factor, and the way the nervous system modulates the heart rate. Therefore, if we want to better better understand how arryhtmias occur, we need to further study and comprehend both cardiac electrophysiology and the autonomic nervous system, which ultimately can lead us to an improvement in clinical treatments of arrhythmic patients.
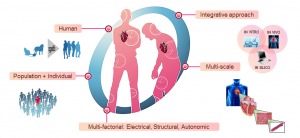
Fig. 1. New computational models can be used to further understand how to deal with cardiac diseases at both individual and population level.
Clinical and experimental analyses have been the main methods used to study cardiac arrhythmias. During the last years, however, computer simulations have established themselves as a new powerful tool to further develop our understanding about the ionic mechanisms that may cause cardiac arrhythmias. By coordinating clinical research and computational experiments focused on the simulation of biological processes (formally known as in silico experiments), it is possible to develop computational models that can help us to unravel and improve the understanding of the different ionic mechanisms linked to arrhythmic risk. In addition, we can use computational models to perform experiments that would not be possible to carry out on animals and people, such as simulations to study the effects of ageing, of different drugs, or even the presence of various diseases.
Consequently, the research in which the BSICoS group (Biomedical Signal Interpretation and Computational Simulation) at the University of Zaragoza is focused on combining clinical and experimental knowledge of cardiac electrophysiology with computational simulation methods to develop personalized models for each patient (Fig. 1). These models are implemented from measurements of the electrical activity of cardiac cells and tissues. Note that these measurements are what we call “action potential signals”, which can be obtained from techniques such as patch-clamp (Fig. 2, left) or optical mapping (Fig. 2, right), and allow us to record the electrical behavior of different samples and biopsies.
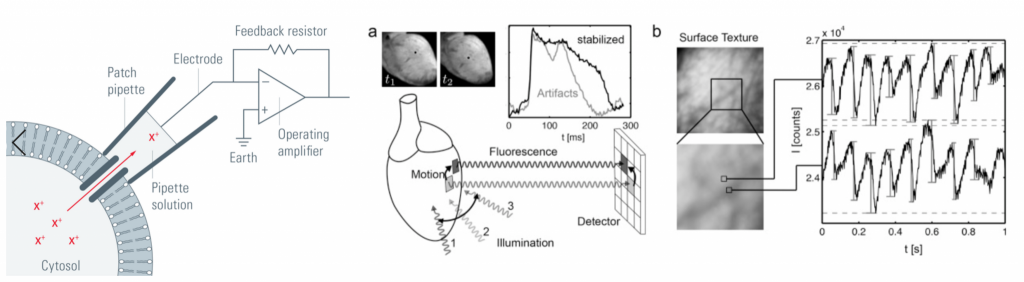
Fig. 2. (left) Functional diagram of the Patch-Clamp technique [2]. (Right). Functional diagram of the Optical Mapping technique [3].
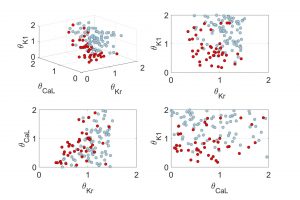
Fig. 4. Example of the results obtained with computer simulations. The combinations of ionic channels together with a greater predisposition to suffer arrhythmias (in disease conditions under stimulation of the nervous system) are coloured in red.
From these electrical signals, and by using mathematical estimation methods such as the Unscented Kalman Filter or the Double Greedy Dimension Reduction [4], we are able to estimate the value of different parameters, and thereby implementing the different individualized models for each patient’s heart. Specifically, the parameters that we want to include in these models, which will eventually determine their behaviour, are the number of different types of ion channels (i.e., sodium, calcium, and potassium) located in the cell membrane. When these ions go through these channels (bidirectionally), an electrical activity is generated and, in turn, the muscle contracts or relaxes, causing the heart to beat. The number of channels varies depending on the area of the heart they are located, and varies throughout life and from one individual to another. At the same time, some diseases can block certain ionic channels, which leads to the development of very dangerous pathologies. Therefore, being able to predict the number of each of type of ion channels is crucial, and this is what we aim to do with our mathematical methods in our research team. Once we have calculated an estimated value for them, we then implement individualized models that can reproduce the experimental signals we observed in the patients’ hearts regarding their morphology and beat-to-beat variability. Besides, with this information, we can also infer values or combinations of values that could lead to a higher risk of arrhythmia (Fig. 4). This can help us to predict the risk each patient has to suffer arrhythmias, ultimately aming to develop personalized guided therapies. Thanks to these personalized models, we could adapt the medical therapy to each individual and check in advance if the prescribed medication could be effective and properly work.
In summary, just as technology has reached both our homes and our pockets, it has come into our hearts too. It is crucial that we acknowledge the importance of all computational and technological advances because, thanks to them, we will also be able to enjoy a healthier and longer life. Therefore, next time you hear that the heart has nothing to do with computers, you will be able to firmly deny it: they are a couple, a strange couple, but a couple after all.
* * *
By David Adolfo Sampedro Puente, PhD student at University of Zaragoza.
More information:
- John RM, et al. (2012)
- Veitinger S, et al. (2011).
- Christoph J, et al. (2017).
- Sampedro-Puente DA, et al. (2019).